Since the introduction of Global Navigation Satellite Systems (GNSS) has shepherded in an era of unparalleled accuracy and reliability in determining precise locations across the world. However, not all GNSS receivers achieve the same level of accuracy. It is important to understand the difference between Real-Time Kinematics (RTK), Differential GPS (DGPS), L-band 10 cm accuracy systems like Hemisphere’s Atlas, and autonomous systems as they can make or break your survey.
RTK stands atop the GNSS accuracy options, relying upon a base and rover tandem to achieve centimeter-level accuracy in real-time. You will most often find RTK receiver in fields where high absolute accuracy is needed, such as land surveying, construction layout, and precision agriculture. In a tier slightly below, DGPS steps in, using additional regional satellites to correct errors in GNSS signals received by the receiver, elevating standard GPS accuracy to sub-meter or decimeter levels. Relying upon clear views of the sky, DGPS is most often used in marine navigation, geodetic surveying, and agricultural machinery guidance where enhanced, though not pinpoint, accuracy is essential. Similarly, L-Band systems, like Hemisphere’s Atlas, leveraging satellite-based augmentation to achieve reliable centimeter-level accuracy globally, amplifying its significance in construction, machine control, and precision agriculture realms. Lastly, autonomous accuracy systems, operating without external correction signals, giving accuracies to within a few meters, catering to applications in consumer-grade navigation, fitness tracking, and general mapping where exact centimeter-level precision may not be critical but reliable positioning remains essential.
- RTK (Real-Time Kinematics)
- DGPS (Differential GPS)
- L-Band Corrections
- Autonomous Corrections
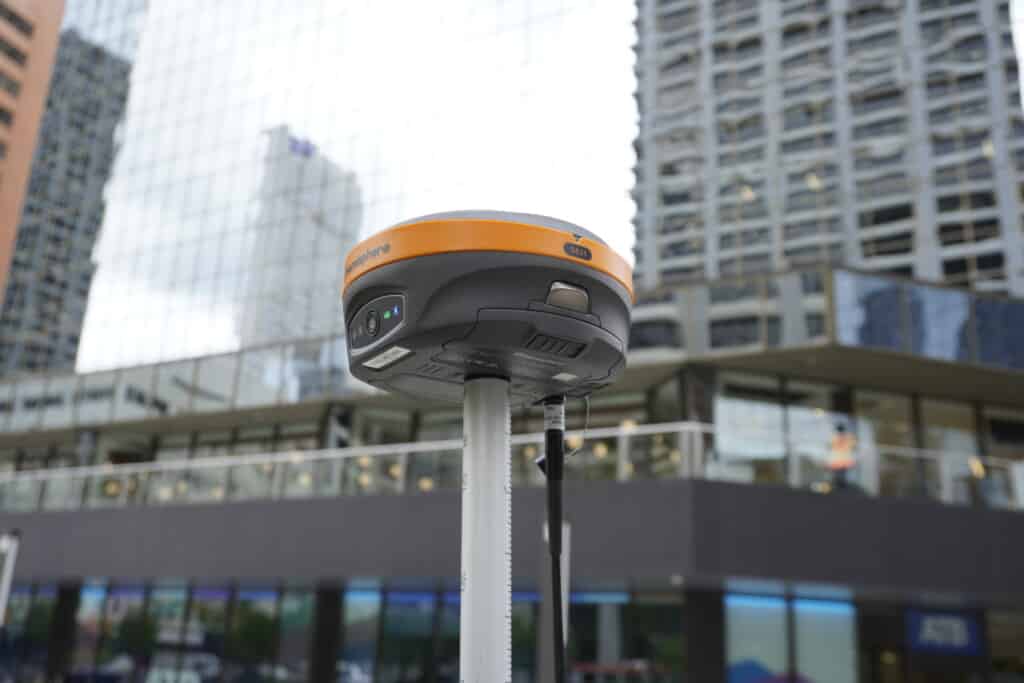
RTK (Real-Time Kinematics)
Real-Time Kinematics is a high-precision satellite navigation technique that stands at the forefront of accurate GNSS positioning systems. Its fundamental principle involves the use of a base station and a rover, both equipped with GNSS receivers. The base station, typically located at a known position, receives signals from satellites in the GNSS constellation. This information is transmitted to the rover where the RTK magic happens.
How RTK Works
Base Station: The base station precisely determines its position by calculating the phase differences of the satellite signals it receives. It continuously monitors these signals and computes corrections for errors caused by factors like atmospheric disturbances and satellite clock drift.
Rover: Simultaneously, the rover, which could be a moving vehicle or a handheld device, receives satellite signals and correction data from the base station in real-time. By integrating the correction information received from the base station, the rover corrects its own position calculations, significantly enhancing its accuracy.
Achieving Centimeter-Level Accuracy
RTK technology enables centimeter-level accuracy by exploiting phase measurements of carrier signals from GNSS satellites. The corrections provided by the base station are transmitted to the rover with minimal latency, allowing for highly precise positioning even in dynamic environments.
Applications of RTK
RTK finds extensive use in applications that demand utmost accuracy, such as:
Surveying and Mapping: Land surveyors rely on RTK for precise measurements in construction layout, topographic mapping, and boundary delineation.
Precision Agriculture: Farmers use RTK for precise guidance of agricultural machinery, allowing for optimal seed planting, fertilizer application, and crop monitoring.
Construction and Engineering: In construction projects, RTK assists in accurate site preparation, grading, and building layout.
Challenges and Limitations
While RTK offers unparalleled accuracy, it is sensitive to signal obstructions and multipath errors, particularly in urban areas with tall buildings or dense foliage. Additionally, the need for a continuous communication link between the base station and rover limits its operational range.
Advancements in RTK Technology
Ongoing advancements in RTK technology aim to mitigate its limitations. Innovations such as multi-constellation support (utilizing signals from multiple satellite systems like GPS, GLONASS, Galileo, and BeiDou), enhanced algorithms for error correction, and integration with inertial measurement units (IMUs) are expanding the capabilities and robustness of RTK systems.
The latest 7th generation RTK receivers like Hemisphere’s S631 can achieve accurate positioning even in difficult environments where signals are less than ideal. Such as those found under trees and near buildings. Check out this video where we tested the repeatability of the Hemisphere S631’s RTK positioning under trees.
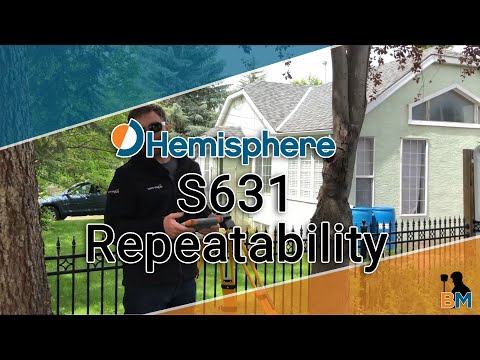
Real-Time Kinematics remains a cornerstone of precision positioning, continually evolving to meet the demands of various industries requiring centimeter-level accuracy in dynamic environments.
DGPS (Differential GPS)
Differential GPS serves as a practical and cost-effective method to enhance the accuracy of standard GPS systems. It operates on the principle of comparing the signals received from GPS satellites at a stationary reference station with those received at a mobile unit, correcting for errors and significantly improving positional accuracy.
How DGPS Works
Reference Station: A stationary reference station with a known location continuously receives signals from GPS satellites. This station precisely calculates its own position and simultaneously measures any errors in the signals it receives.
Mobile Unit: The mobile unit, which could be a vehicle or handheld device, receives signals from the same satellites but experiences similar errors due to atmospheric conditions and signal disturbances.
Error Correction: By comparing the signals received at the reference station with those received by the mobile unit, DGPS calculates and transmits correction data to the mobile unit, effectively mitigating errors caused by factors like ionospheric and tropospheric effects, satellite clock drift, and ephemeris errors.
Achieving Improved Accuracy
DGPS significantly enhances GPS accuracy, reducing positional errors from the standard GPS accuracy of several meters down to sub-meter or decimeter levels (in the case of Galileo’s new HAS service). This level of accuracy makes it suitable for applications where high precision, but not necessarily centimeter-level accuracy, is required.
Applications of DGPS
Marine Navigation: DGPS is extensively used in maritime operations for precise vessel positioning, aiding in safe navigation, docking, and offshore operations.
Geodetic Surveying and Mapping: Surveyors and cartographers utilize DGPS for accurate mapping, land surveying, and geodetic applications where moderate accuracy is sufficient.
Agricultural Machinery Guidance: In farming, DGPS assists in guiding machinery for planting, spraying, and harvesting, optimizing field operations.
Challenges and Considerations
While DGPS offers improved accuracy, it requires a reference station at a known location within range of the mobile unit. This limitation restricts its use in areas were establishing or maintaining a reference station network is challenging, such as remote or sparsely populated regions.
Advancements and Integration
DGPS continues to evolve with advancements in technology. Integration with other positioning techniques, such as RTK and augmentation systems like SBAS (Satellite-Based Augmentation Systems), extends its capabilities and coverage, overcoming some of its limitations.
Commonly, DGPS receivers are used in GIS applications. Receivers like Juniper’s Geode are perfect for applications where sub-meter accuracy is desired, while keeping the receiver as small and compact as possible.
Differential GPS stands as a reliable solution for achieving higher accuracy in positioning systems, offering a balance between precision and cost-effectiveness across various industries and applications.
L-Band 10 cm Accuracy (e.g., Hemisphere’s Atlas)
L-band technology, epitomized by services like Hemisphere’s Atlas, represents a significant stride in augmenting Global Navigation Satellite Systems (GNSS) accuracy to achieve reliable centimeter-level precision globally. By harnessing satellite-based augmentation systems, L-band services offer a substantial leap in accuracy, catering to industries reliant on precise positioning for optimal operations.
How L-Band Technology Works
Satellite-Based Augmentation: Hemisphere’s Atlas utilizes satellite-based augmentation systems to enhance GNSS accuracy. These systems employ geostationary satellites to broadcast correction signals, compensating for errors present in GNSS signals received by the user’s receiver.
Correction Signals: These correction signals contain precise data that mitigate errors arising from factors such as ionospheric disturbances, satellite clock deviations, and orbit inaccuracies, enabling the user’s receiver to refine its position calculations.
Achieving 10 cm Accuracy Globally
Hemisphere’s Atlas, among other L-band services, ensures a consistent, centimeter-level accuracy on a global scale. By amalgamating data from multiple GNSS constellations like GPS, GLONASS, Galileo, and BeiDou, and employing sophisticated correctional algorithms, this technology transcends geographical boundaries, delivering reliable positioning information with unprecedented precision.
Applications and Industries Benefiting from L-Band 10 cm Accuracy
Construction and Machine Control: In construction and heavy machinery operations, where precision is paramount, L-band technology aids in precise grading, excavation, and machine guidance, optimizing efficiency and reducing rework.
Precision Agriculture: Farmers leverage L-band services for accurate crop monitoring, variable rate application of fertilizers and pesticides, and autonomous machinery guidance, enhancing productivity and resource management.
Surveying and Infrastructure Development: Surveyors and engineers rely on L-band accuracy for precise mapping, land development, and infrastructure construction, ensuring accuracy in design and implementation.
Limitations and Future Advancements
While L-band technology significantly enhances accuracy, it may face limitations in heavily obstructed environments or areas with signal interferences. Continuous advancements in satellite technology, integration of additional constellations, and refined algorithms aim to overcome these limitations, further bolstering the reliability and coverage of L-band systems.
Hemisphere’s Atlas and similar L-band services stand as pillars of accuracy, empowering various industries with the precision necessary for critical operations, fostering efficiency, and elevating productivity while setting new benchmarks in global positioning accuracy.
Autonomous Positioning
Autonomous positioning refers to the use of GPS/GNSS receivers without external corrections or augmentation systems to determine the user’s position. Unlike RTK or DGPS, which rely on additional data for error correction, autonomous systems work solely based on signals received directly from satellites.
How Autonomous Positioning Works
Satellite Signals: GPS/GNSS receivers capture signals transmitted by satellites orbiting the Earth. These signals contain information used to calculate the receiver’s position based on the distances from multiple satellites.
Triangulation: Using trilateration or multilateration techniques, the receiver determines its position by calculating the intersection of spheres or hyperboloids representing distances from each satellite.
Error Sources: Autonomous positioning faces inherent errors due to factors like atmospheric disturbances, signal reflections (multipath), satellite geometry, and clock inaccuracies. These errors typically result in accuracy within a few meters.
Achieving Moderate Accuracy
Autonomous positioning systems offer moderate accuracy, typically within a range of 1 to 3 meters. This level of accuracy suffices for many everyday applications where precise centimeter-level details aren’t critical.
Applications of Autonomous Positioning
Consumer Navigation: GPS devices in smartphones and car navigation systems use autonomous positioning to provide users with accurate location information for directions, mapping, and location-based services.
Fitness and Activity Tracking: Wearable devices equipped with GPS/GNSS capabilities track outdoor activities, providing users with data on distance covered, speed, and routes.
General Mapping and GIS: Autonomous positioning aids in general mapping applications, geographic information systems (GIS), and location tagging in photography and social media.
Limitations and Considerations
Limited Precision: The accuracy of autonomous positioning systems, while sufficient for many applications, falls short in scenarios that demand centimeter-level precision.
Vulnerability to Signal Blockage: Signal obstructions caused by tall buildings, dense foliage, or indoor environments can degrade accuracy or even disrupt satellite signal reception, impacting position determination.
Potential for Error Accumulation: Over extended periods or long distances, autonomous systems may accumulate errors, affecting the accuracy of continuous tracking.
Advancements and Future Trends
Advancements in GPS/GNSS technology, including the integration of multiple satellite constellations (e.g., GPS, GLONASS, Galileo, BeiDou) and improved receiver algorithms, aim to enhance the accuracy and reliability of autonomous positioning systems. Additionally, developments in sensor fusion with inertial measurement units (IMUs) contribute to better positioning accuracy, especially in challenging environments.
Autonomous positioning systems serve as the backbone for everyday location-based applications, offering reliable accuracy suitable for a wide range of consumer and commercial purposes.
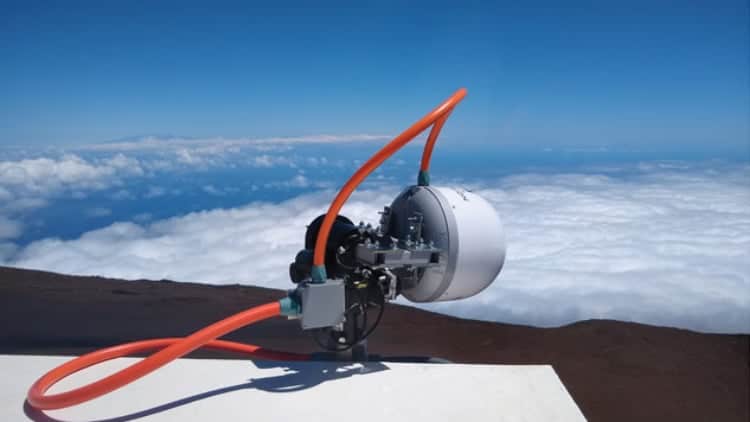
Conclusions
In the realm of Global Navigation Satellite Systems (GNSS), varying accuracy levels cater to distinct needs. Real-Time Kinematics (RTK) provides centimeter-level precision crucial in surveying and precision agriculture. Differential GPS (DGPS) enhances standard GPS accuracy for applications like maritime navigation and geodetic surveying.
L-band technology, exemplified by Hemisphere’s Atlas, delivers reliable centimeter-level accuracy globally, benefiting construction, machine control, and precision agriculture. Autonomous positioning offers moderate accuracy within a few meters, serving consumer navigation and everyday applications.
Advancements, including multi-constellation integration and improved algorithms, promise expanded accuracy and coverage. Understanding these GNSS accuracy levels empowers users to select optimal technology for diverse needs, shaping progress and innovation across industries.
Bench Mark Equipment & Supplies is your team to trust with all your surveying equipment. We have been providing high-quality surveying equipment to land surveyors, engineers, construction, airborne and resource professionals since 2002. This helps establish ourselves as the go-to team in Calgary, Canada, and the USA. Plus, we provide a wide selection of equipment, including global navigation satellite systems, RTK GPS equipment, GNSS receivers, and more. We strive to provide the highest level of customer care and service for everyone. To speak to one of our team today, call us at +1 (888) 286-3204 or email us at [email protected]